Albatross Betting
- Albatross Betting Rules
- Albatross Betting Meaning
- Albatross Butterfly Razor
- Albatross Betting Game
- Albatross Betting Tips
Created by Maj Russ Erb, reviewed by Rich Sugden
NOTE: This page has extensive large graphics, as the primary target audience is the students at the USAF Test Pilot School, who have a high-speed Internet connection to view it with. Anyone is welcome to look at this page--just be patient if you a viewing this over a modem...
Introduction
Cockpit and Fuselage
Aerodynamics and Flight Controls
Propulsion
Landing Gear
Water Adaptation
Other Cool Stuff
Your Host
Introduction
Albatross Golf Resort, Czech Republic. August 26-29 Omega European Masters Crans-sur-Sierre GC, Switzerland. September 2-5 Italian Open Marchco Simone GC, Italy. September 9-12 BMW PGA Championship (Rolex Series) Wentworth (West Course), England. September 16-19 KLM Open Bernardus Golf, Netherlands. September 24-26 Ryder Cup Whistling Straits, USA. 4th Round Any Eagle or Albatross Live - Arnold Palmer Invitational 2021 - Any Eagle or Albatross; Show All. (as long as betting is still available on the market). TOP CASINO GAMES. Irtoto Promotions FAQ 24/7 Support. Sports Results Live-Results Statistics.
The Albatross, the fifth amphibian built by Grumman Aircraft for the military, was designed in the late 1940's for Air/Sea search and rescue, air ambulance, antisubmarine patrol, cargo, and transport. The first of about 450 aircraft built entered service in 1949. The Albatross served with the U.S. Navy, Coast Guard, and the military services of 17 foreign countries. The last aircraft left Navy service in 1976, and some Albatrosses are still in service abroad.
The Albatross usually carried a crew of seven, including pilot, co-pilot, radio operator, radar operator, navigator and two crewmen. The search radar was said to be sensitive enough to spot a liferaft on the open ocean. During the Korean war 900 airmen were rescued by Albatross crews. It has a wingspan of 85 feet, a length of 62 feet and weighs 22,000 pounds empty. It can carry 1700 gallons of fuel in its main, float and drop tanks and has a range of over 3,000 miles. It has two nine cylinder supercharged Wright 1820-76 radial engines that produce 1450 horsepower each and give it a cruise speed of close to 200 mph and a service ceiling of over 25,000 feet. N3HU is an 'A' model Albatross. The 'B' model was designed later, has a longer wingspan, that gives it a greater range and service ceiling, but slightly slower speed. A 'tri'-phibian version with skis was designed to operate off the ice and snow in the polar regions.
Grumman Albatross, USN Bureau Number 131906, was delivered to the Seven Rivers Naval Air Station, Annapolis Maryland on June 23rd, 1953. It subsequently served at NAMC R&D in Philadelphia, FASRON 110, N.A.S. Kwajalein, N.A.S. North Island San Diego, N.A.S. Pensacola, N.A.S. Trinidad, and finished its operational duty at N.A.S. Key West, Florida. On May 9th, 1968 it was ferried to Davis Monthan Air Force Base, Tucson, Arizona for mothballing and storage. It, along with 29 other Albatrosses, was traded for by a Florida aviation enthusiast in the 1980's and Dr. Sugden selected and purchased it in 1993. The aircraft had only 3500 hours of flight time, and underwent complete inspection and replacement (IRAN) overhaul only 700 hours prior to her retirement. It was restored to flying condition by Chuck Wooten of Specialized Aircraft Maintenance in Tucson, ferried to Aerocrafters Aviation Inc. in Santa Rosa California in 1993, and the restoration process was largely completed by the spring of 1995. With the exception of modern avionics and instruments, the interior and exterior were generally restored to original condition and configuration. The original medical stretchers are used as bunks. A marine lavatory replaced the spartan Navy head and a small galley was added.
The aircraft will be used for exploring lakes and territories in the far reaches of Canada, Alaska and eventually the owner hopes to return her to the South Pacific. N3HU is based in Driggs, Idaho and spends many of the summer months on Lake Pend Oreille outside of Sandpoint, Idaho.
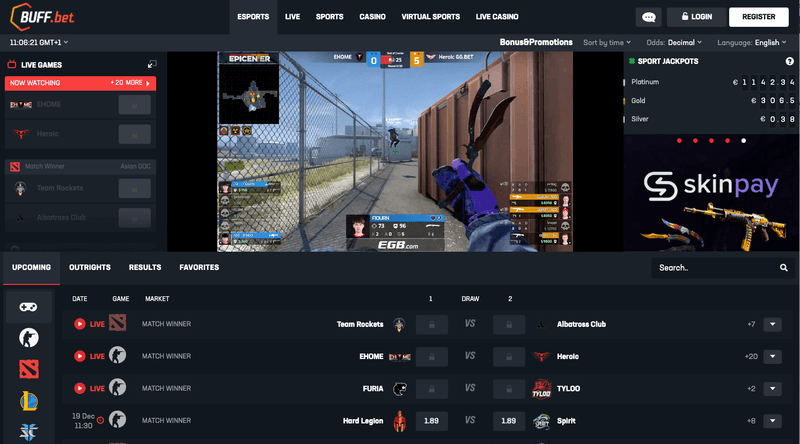
Cockpit and Fuselage
The aircraft is entered through a hatch (no doors-this is a Navy bird) on the left side of the fuselage. Since the hatch has to be above the water line, it requires climbing this ladder to get in. I guess you could try to jump up there, but using the ladder looks much less silly.The door is split horizontally ('Dutch Door'). Opening just the top half provides a higher freeboard in rough weather to keep the waves out. A rescue platform can be attached to the bottom door sill to assist pulling in people from the water.
Just to the left of the door is a retractable step (at the bottom of the black line, above the window) that is used for climbing up to the top of the fuselage.
Just inside the hatch, looking forward, we see the passenger/cargo compartment. This picture does a poor job of relating how big this compartment feels. On the left are four original litters, retained for use as crew rest bunks. Forward on the right are four seats. The hatch at the front of this compartment leads into the cockpit.
Moving to the front of the passenger/cargo compartment and turning around, we see the hatch leading to the lavatory and 'head,' which can be very important on those 16.5 hour sorties.
Looking through another Navy style hatch into the flight deck. The walkway is a the same level as the deck (Navy for floor) in the passenger/cargo compartment, but the flight deck on either side is about kneecap height. Pilot and copilot seats are where you'd expect them, with an additional seat behind the pilot and another behind the copilot.
View of the pilot's side of the instrument panel. A complete set of primary flight instruments is installed on each side of the cockpit. It's a good thing too, since looking cross cockpit is a loooong way away.
On top of the left end of the pilot's yoke is a coolie hat trim switch which was installed by the current owner. Fore and aft movement controls the left elevator trim, much as you would expect. Left and right movement controls the rudder trim, NOT the aileron trim as in many other installations. You'll probably find that this was a good idea, since you'll need rudder trim a lot more than aileron trim as you change your power settings.
A closer view of the pilot's instruments.
Engine instruments, fuel quantity, and gear and flap position instruments are located in the center of the panel between the pilots.
All trim tabs are electrically controlled. The original trim switches for elevator, rudder, and aileron trim located on the center console, along with indicators for the current trim position. The coolie hat switches on the yokes are simply connected to the same circuits as the left elevator and rudder switches shown here. The left and right elevator trim tabs are independent systems, giving redundancy in the pitch axes.
Note also in this picture that the primary engine controls (throttle, mixture, etc) are located on the overhead center console. This was presumably easier to implement since the engines are located on the high wing.
Copilot's flight instruments.
The overhead center console contains the primary engine controls. The throttles and mixture controls are levers as you would probably expect. The propeller RPM is electrically controlled and hydraulically actuated. The two toggle switches are pushed forward to increase RPM, and pulled backwards to decrease RPM. These switches are spring-loaded to center, so you must hold them in the appropriate position until the desired RPM is reached. To learn more about the general operation techniques of these engines, check out the Beech 18 procedures, which are similar.
The supercharger control changes the gear ratio of the engine-driven supercharger. Other than checking operation before takeoff, expect to leave this control in the 'Low Blower' position for the entire flight. The low speed setting is sufficient at low altitudes to provide the required manifold pressure. At high altitudes, where the ambient pressure is lower, the high speed setting is engaged to additionally compress the air before it goes to the engine. At low altitudes, when the low speed setting is sufficient to supply the maximum manifold pressure, engaging the high speed setting actually reduces the power available by the amount of power required to drive the supercharger faster, with no usable increase in manifold pressure, since the manifold pressure is already at the maximum allowable value.
The throttle friction lever can be easily moved to change the friction on the throttle levers. Typically you would want lots of friction in cruise flight, much less for taxi, takeoff and landing where many small throttle movements are required.
The flap control is simply pulled down until the desired flap setting, printed on the side, is visible. Choices are 15, 30, and 40 degrees.
Fuel management is accomplished by turning the fuel selector handle to the desired tank. Expect to leave these on the main tanks for your entire flight. For long range missions, fuel from the auxiliary tanks is normally transferred to the main tanks before going to the engine. If the transfer pumps fail, fuel can be drawn directly from the auxiliary tanks, but no fuel gauges are provided for the auxiliary tanks. You'll know the aux tank is empty when the engine quits. Crossfeeding is possible by selecting the tanks from the opposite wing.
This picture shows the same overhead console behind the fuel selector valves. The red panel has switches required for dealing with emergencies. The two large buttons are for feathering the propellers. Your IP should brief you on how he wants to handle emergencies.
Behind the emergency panel are the various radios, transponders and such. Look for the telephone. Find out how it works.
At the very back of the overhead console is the rudder boost control. Hydraulic rudder boost is normally used during takeoff and landing, and turned off during cruise. Its primary purpose is to relieve pedal forces if required for single engine flight. Evaluate how necessary it is for this purpose and how effective it is. Discuss how you plan to do this with you IP first, of course.
The brakes, flaps, and landing gear on the Albatross are hydraulically actuated. Normally the engine-driven hydraulic pump supplies all the hydraulic pressure you will need. Should this pump fail for some reason, an emergency hand pump is provided next to the pilot's seat. This hand pump can operate any of these systems, depending on where the selector valve (next picture) is positioned.
This hand pump also supplies the hydraulic pressure to set the parking brakes. Hope that this is the only time you'll get to use it, since it is reported that lowering the landing gear takes about 2000 strokes. There's a lot of volume in those landing gear cylinders.
Also seen in this picture is a window in the floor into the nose gear well. Besides allowing close inspection of the nose gear in flight, it also provides a quick cross check during the day if the nose gear (and presumably the main gear) are up or down. Dark window--gear up. Light window--gear down.
The hand pump selector valve has five positions. From the one o'clock position clockwise: Emergency and Parking Brake, Flaps, Landing Gear Down, Engine Pump (as shown), and Landing Gear Up. To move the flaps, use the flap handle (on the overhead console) to set the position and direction of movement. For normal operations, leave the hand pump selector valve in the Engine Pump position.
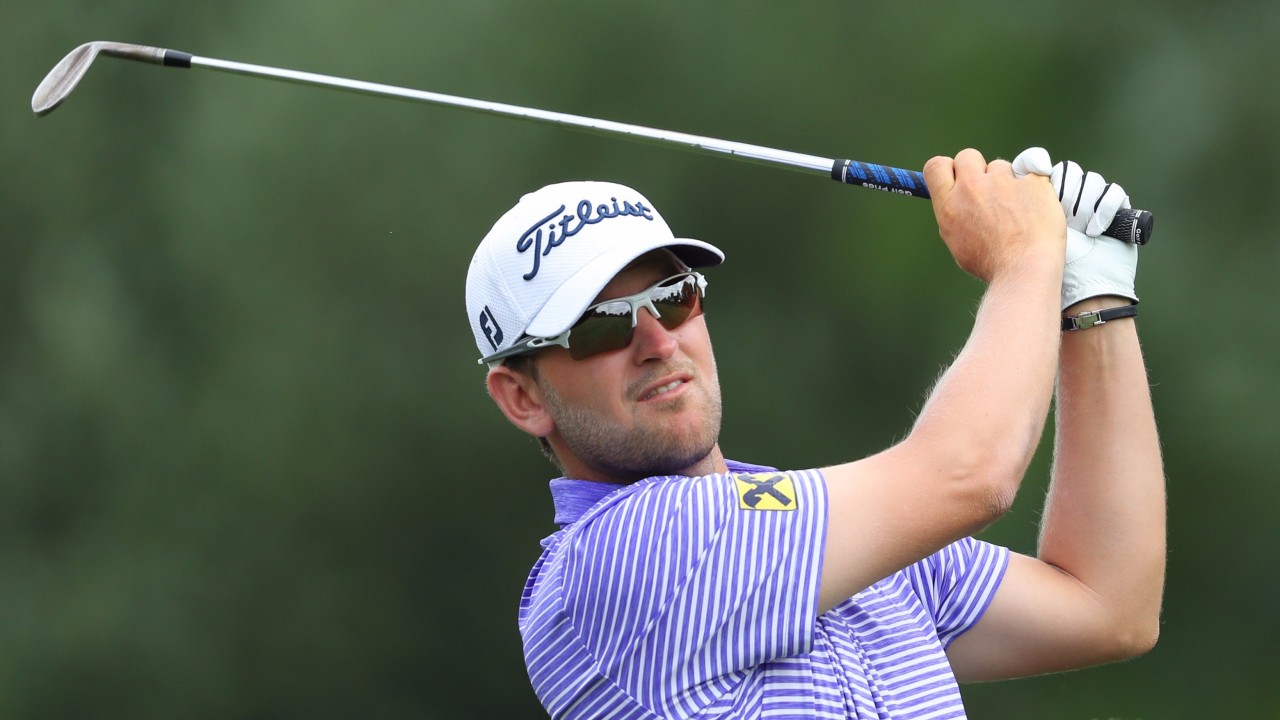
The large red pipe seen here is a control lock for the yoke, and is removed and stored on the back wall of the flight deck during preflight.
Also seen on the right side of this photo is the landing gear handle. For land operations, everything is like you're used to. For water landings, the landing gear must be UP. Landing gear down on the water is just as bad as landing on land with the gear up. Get it wrong and you can bet that the rest of your classmates won't get their qual flights in the Albatross. Besides that, it'll probably ruin the rest of your day.
Immediately above the landing gear handle is the drop tank control panel.
Immediately behind the wing is a large hatch in the roof of the fuselage, with a sextant port in the middle of it. I am told that this hatch was big enough to load an entire R-1820-76 engine quick change kit (engine, mount, and accessories) into the aircraft. We never did figure out how you would get the engine out in the middle of the ocean to fix a crippled aircraft without some sort of crane.
On the back of the sizeable wing spar carry-through structure (guaranteed to instill confidence in passengers) is a handle which, if pulled, starts inflating a life raft on top of the wing. The life raft would push open the hatch as it inflated, then slide down the top of the aircraft to the left side, coming to rest right in front of the exit hatch. During restoration, this handle was accidentally pulled, and the life raft, which had been stored for 20 years, inflated and slid down the left side, just as designed. Since an inflatable boat is carried in the fuselage of this aircraft, the overwing life raft compartment is used to hold engine oil, a line (Navy for rope) to lift the gas hose up to the top of the wing, and other items.
It is possible while loading or unloading the Albatross to get the c.g. behind the main gear, which would result in the airplane unceremoniously sitting on its tail. At the least, it would look awfully funny and your gross buffoonery would be known to everyone around and talked about at the bar for the next 20 years. At the worst, you could damage the aircraft. To prevent this, this tail stand is installed when parked. During preflight, the stand is removed and stored inside the aircraft. Of course, this stand is not required when parked on water.
Bubble windows are installed on both sides of the fuselage. These were originally installed for such purposes as looking for downed aircrews on rescue missions. You simply must experience the thrill of being able to 'stick your head outside the fuselage' without the annoying side effects, such as the slipstream mussing your hair. It's a trip! This is also a great vantage point to watch the main gear retraction and extension, and for watching the 'rooster tail' during water landings and takeoffs. For the photographically inclined you can also get a great shot of the aircraft shadow immediately after liftoff if the sun is in the right position.
An emergency exit is located on the fuselage opposite the entry door. The two hooks in the center of the hatch are attach points for JATO (Jet Assisted TakeOff) rockets. For various reasons of safety, lack of mission requirements, and, of course, cost, JATO bottles are no longer used on this aircraft.
This particular aircraft is equipped with a platform that can hung outside this hatch when open. The owner tells us stories of landing on a lake somewhere, opening this hatch, setting a marine barbeque on the platform (on the lee (Navy for downwind) side, of course), and having a picnic on top of the fuselage!
Which brings up the question of how do you get on top of the fuselage without a ladder in the middle of the lake. Very easily. Simply open the entry hatch (remember it's above the waterline), and standing in the hatch, put your right hand in the first handhold. The handhold is a spring loaded door that can be easily pushed down. Then put your left foot on the retractable step just in front of the entry hatch. Lift yourself up on this step and grab the second handhold with your left hand. Continue to pull yourself up, place your right knee on top of the fuselage, and scramble the rest of the way up onto the fuselage. To get down, reverse the procedure. To find the step (you won't be able to see it), just run your foot down the black line until you hit it.
The darker areas in this photograph are non-skid areas for walking on.
Here your illustrious author has worked his way up onto the top of the airplane and forward to the number 1 engine. If I don't look too comfortable here, it's because the wind was howling at 20 to 30 knots and I didn't want to fall off!
The Pitot tube is located near the right wing tip. Landing lights are located under both wing tips. These lights are hinged at the front edge and rotate down and forward when in use.
A fixed, ground-bendable trim tab is located on the right aileron.
The static ports (orange arrow) are located on both sides of the nose section in the stars and bars insignia.
Aerodynamics and Flight Controls

The elevator and rudder are fabric covered. The condition of the tail is important to check on preflight and postflight since the tail surfaces take quite a beating from water spray during water takeoffs.
Fabric covering was retained on flight control surfaces of many types of aircraft for many years after the rest of the aircraft was covered with aluminum. One of the primary reasons for using fabric was to keep the control surfaces lighter so that less weight would be required to mass balance the surface along the hinge line and to reduce sluggishness in the controls. This was important in reversible control systems. As aircraft design speeds increased, there were problems with strength of the fabric. However, high control forces drove designers to using irreversible control systems, with the actuators rigidly holding the control surface in its proper place. With the rigidity of the actuator, there was no longer a requirement to have the surface precisely balanced, and control surface weight was no longer as important of a consideration. Hence, the fabric could be replaced with metal skins, which held up better to the higher speeds.
Looking aft at the tail. The black leading edges are rubber deicing boots, which can be inflated and deflated to break off accumulated ice in flight.
The ailerons are also fabric covered. Aileron trim is by a trim tab on the left aileron. A fixed tab is provided on the right aileron, which can be used to offset airframe asymmetries.
The flaps are aluminum covered, and are electrically controlled and hydraulically operated. The flaps are also hydraulically balanced, a feature unique to Grumman aircraft. In the absence of air loads, lowering the flap lever lowers the flaps, which may come down asymmetrically. However, as soon as air loads are applied, a cross-feed between the actuators will allow the flaps to re-adjust until the loads, and thus position, are symmetric. To see this, have the pilot set the flaps to 15 degrees down while parked. Watch the flaps through the bubble window. The flaps will be down, but probably not both at 15 degrees. When the aircraft starts moving for takeoff, and possibly even a reasonably quick taxi, the airloads on the flaps will move them into the proper position without touching any controls.
Each wing contains a slot from the tip float out to the wing tip. At high angles of attack, these slots help to keep the airflow attached to the outer portions of the wing so that the inboard portion of the wing will stall first. The benefit of this arrangement is that the ailerons should remain effective up to stall.
The slot is located such that at high angles of attack, high pressure air will be driven into the front of the slot, as shown by the arrows. The cross sectional area of the slot decreases as the air passes through it, additionally accelerating the flow through the slot.
The high speed air exits the top of the slot into the wing boundary layer, as shown by the arrows. This high speed air re-energizes the boundary layer just before it separates, causing it to stay attached.
B model Albatrosses had the slot removed and the leading edge was drooped. A drooped leading edge accomplishes the same effect by presenting the air with a more gentle curvature, thus inhibiting separation.
During your flight, test the stall characteristics of the Albatross and determine for yourself if the slot is effective in maintaining aileron effectiveness up to the stall.
The leading edge of the wing is fitted with a rubber deicing boots, which can be inflated and deflated to break off accumulated ice in flight.
A bomb rack can be seen under the wing between the propeller tip and the word 'NAVY.'
Propulsion
Power is supplied by two nine cylinder two-speed supercharged Wright R-1820-76 radial engines of 1450 horsepower each. Versions of the same engine are used on the T-28 and the B-17.Each engine drives a three-bladed constant speed propeller. Unlike most constant speed propellers, these propellers are electrically controlled and hydraulically actuated independent of engine oil. The governor RPM is set by toggle switches, and the governor and propeller hydraulic pump are driven by the engine. The propeller uses a lighter weight oil than the engine. This system allows immediate control of the propellers on engine start without waiting for the engine oil to warm up. When floating on the water with the engines running, the propellers are thrusting, and the aircraft will move in response. On the water at low speeds, the air rudder is not effective, and no water rudder is installed. The primary means of directional control is differential power. At idle power settings, control is best accomplished by moving one prop in and out of reverse. No beta range was available on these propellers, so the propellers were always in either forward or reverse thrust.
A propeller oil cooler is integrated into the hub dome. The arrows show the flow of air into the propeller oil cooler.
These arrows show the flow of air out the back of the propeller oil cooler.

On the right side of the photo are shown the engine exhaust stacks. Two sets of exhaust stacks on each engine exit on the top side of the nacelle. Placing the exhaust stacks on top of the nacelle helps to protect them from the water spray on takeoff and landing.
These engines are air cooled. Cooling air enters the front of the cowling. Baffles force the air to travel between the cooling fins on the cylinders and cylinder heads. After passing around the engine, the cooling air leaves the cowling through the cowl flaps. The cowl flaps can be moved to vary the size of the cooling air exit, thereby controlling the amount of cooling air through the cowling. Too much cooling air creates additional drag and overcools the engine so that it does not run at its best temperature. Not enough cooling air causes the engine to overheat.
While airflow around the engine sufficiently cools the cylinders, the internal workings of the engine are cooled by the lubricating oil. To cool the oil, it is passed through an oil cooler. This cooler is simply a radiator which air from the cowling that has already passed over the engine flows through to cool the oil. The amount of air passing through the oil cooler is controlled by the position of the oil cooler flap. If the flap is wide open, a large volume of air can pass through the oil cooler, giving more cooling. Closing the flap to a smaller opening allows less air to pass through, giving less cooling.
Radial engines are 'dry sump' engines, meaning that the oil is stored in a tank separate from the engine. Most automobile engines are 'wet sump,' with an oil pan (sump) on the bottom of the crankcase where the oil is stored when it is not being pumped through the engine. In a wet sump engine, the oil pump draws the oil from the oil pan, pushes it through channels into all parts of the engine, where the oil drips off the various parts and works its way back down to the oil pan.
It would not be practical to store the oil in the crankcase of a radial engine, because it would simply flow down into the lower cylinders. To give the oil a place to temporarily drain, skirts which extend up into the crankcase are fitted to the lower cylinders. The oil can drain down the walls of the crankcase, but is prevented from entering the lower cylinders by the skirts, which act something like a dam. A scavenging pump then draws the oil from this area and sends the oil back to the oil tank.
Lesser amounts of oil can still get down into the lower cylinders. While the engine is running, this is not a problem because the oil will be expelled with the exhaust gases. When the engine is not running, however, some oil may collect in the lower cylinders. If this happens, when the pilot tries to start the engine, the piston will try to compress the oil in the cylinder, fail to do so (because liquids are essentially incompressible), and proceed to break things, making very expensive noises. This condition is known as 'hydraulic lock.'
In land aircraft, hydraulic lock is easy to check for and to correct. During preflight, the pilot simply pulls the propeller through at least two rotations (being very careful to ensure that the ignition is off). If hydraulic lock is going to happen, it will happen when the pilot tries to pull the propeller through as the piston in the offending cylinder tries to compress the oil. This will stop the prop. If hydraulic lock is found, it can be fixed simply by removing the spark plugs from the lower cylinders and letting the oil drain out.
On the water, though, it is kind of tough to get out in a boat, paddle up under the props, and then try to turn them over without falling out of the boat (although it might be funny to watch...). As such, after engine shutdown, additional electric scavenging pumps are run for a few minutes to pump as much oil as possible out of the crankcase and send it back to the oil tank. This will greatly reduce the chances of hydraulic lock. Most land aircraft would not have these additional scavenging pumps.
At taxi speeds and while the aircraft is stopped, there is not much air flow through the engines for cooling. As such, the cowl flaps and oil cooler flaps are normally open wide, as seen here, for ground operations to get the maximum amount of cooling air. For takeoff, the cowl flaps and oil cooler flaps are moved to the 'trail' position, which lines them up with the contours of the cowling. The smaller exit area is offset by the higher airspeed of the cooling air, so that the same temperatures can be maintained. The exit area can be further fine tuned in flight by moving the cowl flaps and oil cooler flaps to control engine temperatures.
This view is from the seat immediately behind the pilot, looking out the small circular window.
The outlet of the crankcase breather is located just inboard of the tip float. When this picture was taken, the right engine was losing some oil through the breather, hence the oil trail on the underside of the wing. The left engine was doing better, and did not have an oil trail behind its breather tube.
Fuel can be carried in six different tanks. The main tanks are located in the inboard section of each wing between the fuselage and engine nacelle. The main tanks have a capacity of 338 U.S. gallons each. Drop tanks of 100, 150, or 300 U.S. gallons each can be fitted to the bomb racks on each wing outboard of the engine nacelles. Each tip float is also a fuel tank, capable of carrying 200 gallons each. The fuel filler cap location is shown here. Full use of the tip float tanks is restricted to land operation only, as when these tanks are full of fuel the tip floats don't. Runway landings are also prohibited with more than a 100 gallon difference in fuel load between the tip floats. For water operations, the tip floats must contain less than 100 gallons of fuel each. Therefore, if taking off with a full load of fuel (such as when this aircraft flew from Nome AK to Japan in 16.5 hours), fuel is used out of the tips as soon as possible so as to regain the ability to land on the water if required. Even so, for approximately the first two hours water landings are not possible.
Under normal conditions, each engine draws fuel from the main tank on its side of the aircraft. Fuel from the tip float tanks and drop tanks is transferred to the main tank by a 300 gallon per hour electric pump. When either auxiliary tank is empty, a 'Xfer Pump' light is illuminated, indicating no pressure in the transfer line. The crew then turns off the transfer pump. Fuel can be drawn directly from the auxiliary tanks if the transfer pump fails, but no fuel quantity gauges are provided for the auxiliary tanks.
If necessary to balance laterally or if one engine has to be shut down, the fuel selector can be set to draw fuel out of the tanks in the opposite wing.
Landing Gear
With the high wing necessary to keep the wing, engines, and propellers out of the water spray, not to mention keeping the wing spar out of the cargo compartment, designing a small main landing gear is a challenge, if not impossible. In the case of the Albatross, the main gear is connected to the major structure of the wing and side of the fuselage.The horizontal silver bar near the wheel is one of the two hydraulic actuators used for retracting the main landing gear. The silver portion of the vertical strut is part of the shock absorbing oleo.
This 'knee' in the middle of the vertical strut is a key player in the landing gear mechanism. As shown here, it provides the over-center joint which locks the landing gear in the down position. It is not obvious in this photo, but on the aircraft you can see the micro-switches that actually tell the instrument to indicate that the gear are down and locked.
The small hydraulic cylinder shown here has the primary purpose of forcing this joint over-center (by expanding) on gear extension and of unlocking the joint (by contracting) on gear retraction. A short study of the arms on the aircraft should reveal how this is done.
When the main landing gear retracts, the wheel ends up in the side of the fuselage with the struts running up the side of the fuselage and along the bottom side of the wing, as shown here by the orange lines. After the small hydraulic actuator 'breaks' the over-center joint in the vertical strut, the lower actuator does the majority of lifting the gear into position.
Due to the immense volume of the hydraulic rams on the landing gear and the limited volumetric capacity of the hydraulic pump, the landing gear do not retract simultaneously. The nose gear (with the smallest volume requirement) comes up first, then one of the main gear, then the other. Evaluate if any resulting aircraft reactions are objectionable.
If you can, take the opportunity to watch the main gear retraction and extension from a bubble window just behind the main gear. If you enjoy mechanisms and seeing how things work, this one is a real treat! It's amazing how such a complex folding can be done with just two actuators. See how long the main gear wheels keep turning after retraction.
The limit speed on the landing gear is 140 KIAS.
Hydraulic brakes are fitted on each main wheel. The various hydraulic lines seen in this photo are for the brakes and for the landing gear extension/retraction cylinders.
The main gear uplock is shown in this picture. This hook holds the main landing gear in the retracted position. If this lock failed to release during the normal gear extension sequence, the flight manual stated that a crew member should locate a certain point on the cabin interior, measure down and back a certain amount, and then punch a hole through the side of the fuselage with the crash axe. This would open a hole that that crew member could reach through and manually release the uplock. For some reason, many Navy crew chiefs found this to be a rather inelegant procedure, so an emergency access panel (shown here) was added to virtually all Albatrosses in the field.
If you don't think this is important, consider this: During one of our qual flights, a main gear failed to extend completely due to a hydraulic leak. The emergency access panel was removed, and a pole stolen from one of the stretchers in the cabin was pushed out the hole. With one crew member watching out the bubble window and two other crew members holding the pole, the pole was guided into position to push the landing gear vertical strut into position over-center, thus locking the gear down. A safe landing was made following the incident. Subsequently, the hydraulic leak was fixed and many more flights were made without incident.
The nose gear lowers through doors in the center of the forward part of the hull. Damage to these doors can occur if the aircraft if landed on the water in a too nose-low attitude.
True to Navy tradition, the nose gear has two wheels. I suspect this was more to save space in the lower fuselage by using smaller wheels than for straddling a catapult track. The nose gear is free castering with no nose wheel steering is provided. Directional control during land taxi is provided by differential thrust, augmented by differential brakes (which are generally ineffective for water operations). Evaluate the ease or difficulty of maintaining a precise taxi direction using these systems.
The nose gear compartment is sealed from the hull, and is partially flooded during water operations. A small window in the top of the compartment is visible from the flight deck, giving a quick check if the nose gear is up or down (doors closed or open), at least for daytime operations.
Water Adaptation
Evaluations of the water handling characteristics of the Albatross are made at Lake Isabella. This photo shows the lake over the forward edge of the nose hatch.You will probably fly a box pattern over the lake before landing. On initial, fly low enough to identify any hazards to landing, such as stumps, floating debris (makes a nasty dent when hit at 50 knots), boats, people, etc. To determine the wind direction, look at which way the waves are moving. You can also look around peninsulas for 'slack water,' which is the smoother water on the leeward (Navy for 'downwind') side. Knowing the wind direction and having cleared the landing area, fly a normal downwind, base, and final. On final, stabilize on the desired airspeed and power setting. If the water is not glassy (it's tough to judge altitude over glassy water, not a problem if waves are present), then flare as normal. Be sure to maintain the proper pitch attitude (generally a little nose up) throughout the landing all the way until you are just driving a big boat.
This is a good time (assuming you're not the current helmsman) to go forward to the bow. Back down onto that walkway between the pilot and copilot, duck under the instrument panel, turn on the light, and open the bulkhead hatch. This hatch leads to the nose compartment, where you open the overhead hatch, then stand up in front of the cockpit. Turn around and wave at your pilot. Then plug your headset back in so she can hear you. The primary purpose of this hatch is so you can use a boat hook (long pole with a hook) to grab the mooring buoy, which, of course, is out of the pilot's field of view.
If you look forward, the nose disappears from your field of view. As such, you can spread your arms, tilt your head back, and do your best Kate Winslet impression. Of course, you'll be a lot closer to the water than she was on the Titanic, but hey, think of it as flying low level.
Here you can see the mooring cable attached to the fuselage below the radome. The cable then goes up around both sides of the radome and is attached to the fuselage again for storage in flight. The upper end is accessible to the crew member standing in the nose hatch. The cable is detached at the upper end, taken from around the radome, and then attached to the mooring buoy.
Low speed taxiing (ploughing, or plowing if you prefer) is accomplished with the engines near idle thrust. This still gives a good 5 to 10 knots, much like bringing a motorboat into a dock. Speed and directional control is by the engines. A very effective way to control direction at low speeds is simply to put the prop on the side you wish to turn toward into reverse. Pull the throttle to idle, then push up. Evaluate how easy it is to maintain directional control. Be sure to try it also in a direction that is not directly into the wind to evaluate how wind affects the directional control.
On the left side of the photo you can see that even with the engines near idle a noticeable amount of water spray can come up. Don't go up front if you're not ready to get a little wet!
The tip floats, besides being extra fuel tanks, are used for lateral stability on the water. At low speeds, one tip float will be in the water.
Step taxiing is preferred if you have a long way to go, such as getting back to the takeoff point. This maneuver consists of getting up enough speed to plane across the water ('on the step'), but not enough to take off. Flaps are left in the UP position, which for some reason allows the aircraft to get up on the step easier. Again, the primary job of the pilot is attitude control. Ailerons are used to get the tip floats out of the water (harder than it sounds). Evaluate how easy it is to keep the tip floats out of the water. Don't forget to pull all the way back on the yoke to keep the nose up while applying power. Lead with the left throttle to counter p-factor until the rudder becomes effective. As the aircraft comes up on the step, it will probably start to porpoise (oscillate in pitch), which is not a good thing because this is an Albatross, not Flipper! Stop the porpoising by smartly pulling back on the yoke as the nose is going down. Once the porpoising stops and you are on the step, the pitch attitude becomes more stable. Now you've got a big, high-speed motor boat. Directional control is by rudder at this point. Evaluate the pilot workload during step taxi.
Also evaluate step taxiing downwind. Determine if the reduced airspeed (due to the tail wind), changes the aileron effectiveness. Also consider the effects of the tail wind on the rudder and directional control.
The view forward from the bubble window during a low speed taxi. The right main wheel can be seen on the left side of the photo in the up position.
Water takeoffs start off just like step taxiing, with a few changes. This time the throttles are advanced to full power, and the aircraft transitions from low speed taxi to step taxi. Once on the step, the flaps are dropped to 15 degrees, and at 80 KIAS, pull back and lift off. Once off the water, its just back to being an airplane.
The takeoff can be rather spectacular to watch from the bubble side window. Applying takeoff power sets up an incredible rooster tail, with water going through the lower part of the prop, up the side of the fuselage, and across the horizontal tail, which doesn't do wonderful things for the fabric covered elevators and rudder. Initially, this water plume fully engulfs the bubble window, but eventually the window clears and gives a spectacular view to the rear.
Try to conceive how much power it takes to move a wall of water like this at 50 knots or so. That's why the engine power required for takeoff (2900 HP available at sea level) is so much greater than that required for cruising flight. This should also give you an idea of why the Navy was so interested in fitting JATO bottles to their flying boats to shorten the takeoff runs.
It's awfully tough to carry work stands or ladders along with you and even tougher to set them up on the water. As such, the panel shown is hinged at the bottom to swing out to level to provide a platform to stand on while working on the engine. These work platforms are provided on both sides of both nacelles. Additionally, all cowling parts are connected to the nacelle by cables, so that they can all be removed and retained without taking a swim with the fishies.
No attempt is made to seal the main gear wells. These main gear wells are on the outside of the watertight hull, and during water operations, they are flooded with about 1000 pounds of water. Of course, there is no good reason to keep all of this extra non-useful weight on board while flying. The acceleration of the takeoff run forces most of the water through this hole in the rear bulkhead, where it goes down a tube toward the center of the hull...
...and comes out here. An identical drain is installed on the opposite side of the fuselage.
Albatross Betting Rules
The sharp discontinuity in the bottom of the hull is known as the 'step.' This was first invented by Glenn Curtiss, and is necessary for water takeoffs. The sharp discontinuity causes cavitation of the water and the resulting turbulence breaks the 'suction' of the water that would otherwise try to prevent the aircraft from lifting off. Consider the bottom of the hull as an inverted airfoil. The water flowing over the hull creates 'lift' in the downward direction, just like air over a wing would. The step is equivalent to a spoiler to reduce this downward 'lift.' Without the step, it would be virtually impossible (or at least much more difficult) to take off from the water.
You may notice that the underside of the fuselage is assembled with round head rivets, while the remainder of the aircraft is flush riveted. As told to us by the owner, the Albatross was originally designed and built with flush rivets on the underside. After an operational accident, a large hole in the underside of an Albatross was repaired in the field, but the mechanic only had round headed rivets available. Much to their surprise, the water takeoff performance improved, getting off the water 5 to 10 knots sooner. Word was sent back to Grumman, who verified this with testing. Therefore, all subsequent Albatrosses were completed with round headed rivets on the hull bottoms. In an odd twist to the story, because the sheets were already dimpled/countersunk, they used a special rivet which was essentially a flat head rivet, but with a round head added on top of it. Countersunk round head rivets--only on the Grumman flying boats...
The remainder of the water that didn't escape the main gear well through the main drain hole during takeoff escapes through this smaller hole at the bottom of the well. Of course, on landing, this is where most of it gets in.
Over the ocean, VHF and UHF radios do not have sufficient range to allow communications back to land bases. Therefore, an HF radio is installed on all aircraft that normally operate over the oceans, such as military airlifters and airliners.
Because HF is at a lower frequency than VHF or UHF, it has a longer range, but it also requires a very large antenna. Some aircraft install the antenna between the top of the fuselage and the vertical tail. However, this location would get in the way of operations on top of the aircraft while floating in the water and prevent easy use of the cargo loading hatch on top of the fuselage.
Instead, the Albatross uses a trailing antenna. The lead weight seen here is attached to a long wire antenna that can be let out behind the aircraft in flight. While this works well, the antenna would probably be damaged and cause much damage (how'd you like to be hit by a 10 pound chunk of lead at 80 knots?) if it was still deployed during landing, not to mention the extreme embarrassment. Therefore, to guard against pilot gross buffoonery, the antenna deployment/retraction system is connected to an air pressure switch, which will only let it be deployed above a certain airspeed, and automatically retracts the antenna below a certain airspeed.
Other Cool Stuff
Here is a picture of Albatross N3HU as it looked in the Davis-Monthan boneyard prior to restoration. As you can see, it's really come back a long way.Albatross Betting Meaning
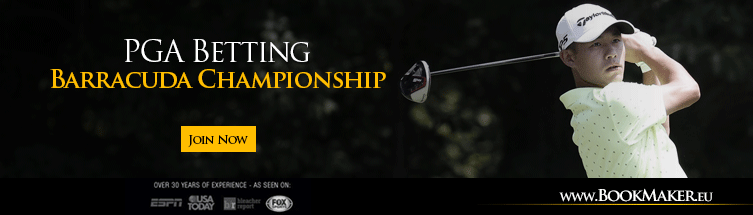
Albatross Butterfly Razor
Your Host
Your friendly Albatross host and instructor pilot is Rich Sugden, M.D., seen here refueling the right main tank. Rich is a Director of EAA Warbirds of America.Albatross Betting Game
EAAChapter 1000 Home PageE-Mail: Web Site Director Russ Erbat erbman@pobox.com
Albatross Betting Tips
URL: http://www.eaa1000.av.org/pix/albatross/albatross.htm
Contents of The Leading Edge and these web pages are the viewpointsof the authors. No claim is made and no liability is assumed, expressedor implied as to the technical accuracy or safety of the material presented.The viewpoints expressed are not necessarily those of Chapter 1000 or theExperimental Aircraft Association.
Revised -- 21 November 2002